Chain Polymerization types
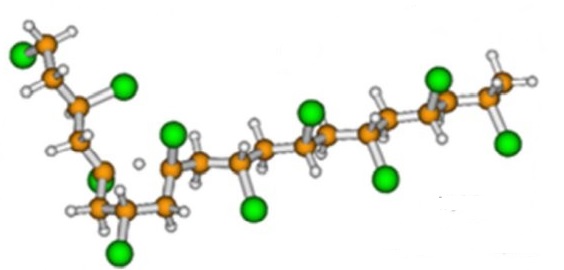
Free radical polymerization has been studied and used extensively for decades. Free radicals are the key components in this polymerization type. Free radical sare highly unstable structures with just one free electron that usually comes from a homolytic breaking of a covalent bond. Usually, a covalent bond is broken in the presence of an initiator that provides the necessary energy or activation energy reduction to induce the homolytic bond breaking. When one electron is found in a free radical, it causes very high reactivity towards third bodies and especially towards structures with shared electrons such as double bonds.
In general, one could describe the free radical polymerization process in three steps. Initially, a free radical is generated from the homolytic cracking of a covalent bond. As discussed, the homolytic cracking can occur due to an initiator but also due to radiation by α, β, γ, or X rays. Free radicals are generated in pairs, in the following fashion:
B2 à B• + B•
In a second step the free radical attacks one or more species [and this is the major disadvantage of free radical processes] and creates a stable structure and a new free radical as in the following equations:
B• + CD à BC + D•
B• + CE à BE + C•
B• + EF à BF + E•
It becomes clear that a free radical is so reactive that the actual product profile of a process could be quite heterogeneous. In order to minimize undesired reactions, very pure mixtures are to be used and termination schemes to be highly effective. The known affinity of free radicals towards double bonds minimizes this effect in free radical polymerizations.
During this second step the polymeric chain continues to grow by adding monomers to its end. At each step the polymeric chain radical increases in size and the single electron is located at its end [exceptions can be found depending on the monomer structure. Examples will be discussed in forthcoming posts]. The propagation step can be described in this case as following:
R• + CH2=CXZ à RCH2-CXZ•
RCH2-CXZ• + CH2=CXZ à RCH2-CZX-CXZ•
RCH2-CZX-CXZ• + CH2=CXZ à RCH2-CZX -CZX-CXZ• … and so on
The last step in this process is called the ‘termination’ step. In this step two free radicals are joined and thus become a new, inactive compound. Termination could also occur with the transfer of the single electron to a third compound [impurities]. In this step we can have a chain length increase higher than the single monomer addition that occurred in step 2. This can be shown as:
RCH2-[CZX]n-CXZ• + RCH2-[CZX]m-CXZ• à RCH2-[CZX]n+m+1-CXZ
Free radical polymerization is a widespread in the polymer and plastics industry.
Anionic polymerization is a well known polymerization approach, both for the lab and industrial scale. The main attribute is the anion or electron attachment to a monomer that leads to the production of a carbo ion. Propagation of polymerization takes place via the consecutive addition of monomers to carbo ions that lead to the production of longer entities, polymeric anions. During anionic polymerization a positive element is always present to achieve local electronic neutralization. It is important to understand however that the presence of the positive element does not lead to an actual reaction with the negatively charged chain end and thus it does not lead to polymerization termination. Commonly this happens [termination] when a quite long chain transfers its activity [negative charge] to a third compound that can be a trace of impurities or even a specific polymerization terminator designed for this purpose.
When the polymer chain grows in very pure environments, it can theoretically continue to grow as long as monomer is still present or continuously supplied. This attribute is commonly referred to as a ‘living polymer’. In that case only thermodynamics can inhibit and terminate the growing of the polymeric chain. This termination can be predicted via investigation of the polymerization reaction system and the relative activation energies of each sub reaction. Gibbs energies of formation for polymeric chains of different sizes show the equilibrium point of such ‘pure’ polymerization processes. Other known termination possibilities include the presence of water or carbon dioxide. Water leads to a saturated polymeric chain with the simultaneous generation of hydroxyl anions.
Substituents of the initial monomer play the most important role regarding the polymerization type of a known monomer. Electrophiles and unsaturated groups lead to stability of the formed anion and are commonly chosen for this polymerization type. On the other hand, the positively charged ‘stabilizer’ or ‘catalyst’ should be a strong electron donor. Different donors lead to different polymerization routes ranging from linear polymeric chains to star shaped polymers. This effect is to be examined in a forthcoming post.
In general, anionic polymerization is preferred for the manufacturing of stereo canonical polymers and high crystalline polymers. Both of the cases are favored by the polymerization process that is strongly affected by the electrostatic forces exerted in the environment of the polymeric chain growth [chain end]. Block polymers are also commonly prepared via an anionic polymerization approach for the same reasons.
Cationic polymerization bears similarities and differences to anionic polymerization. As in anionic polymerization it also is based on a growing carbo ionic chain [carbo cation] that grows by addition of monomers. Catalysts for the cationic polymerization include Lewis acids, protic acids and Friedel Craft catalysts. Lewis acids are commonly used in conjugation with a protic catalyst. As in the case of anionic polymerization, the negative charge remains in the vicinity of the positive charge but without reaction with it. Termination of the polymerization process can occur via different paths. One such possibility is the removal of the end chain proton that leads to regeneration of the catalyst. A rare but not impossible case occurs when the chain cation is so stable that the progress of the reaction becomes unfavored due to thermodynamic limitations.
Cationic polymerization is a very reactive progress; due to this fact, it can often lead to branched polymeric chains. In addition to that, it can proceed even at low temperatures; even as low as -100C at some reported cases. Very low impurities content is tolerated during cationic polymerization due to the fast transfer of reactivity to any molecule.