thermal neutron trapping in Fullerenes; a computational chemistry investigation
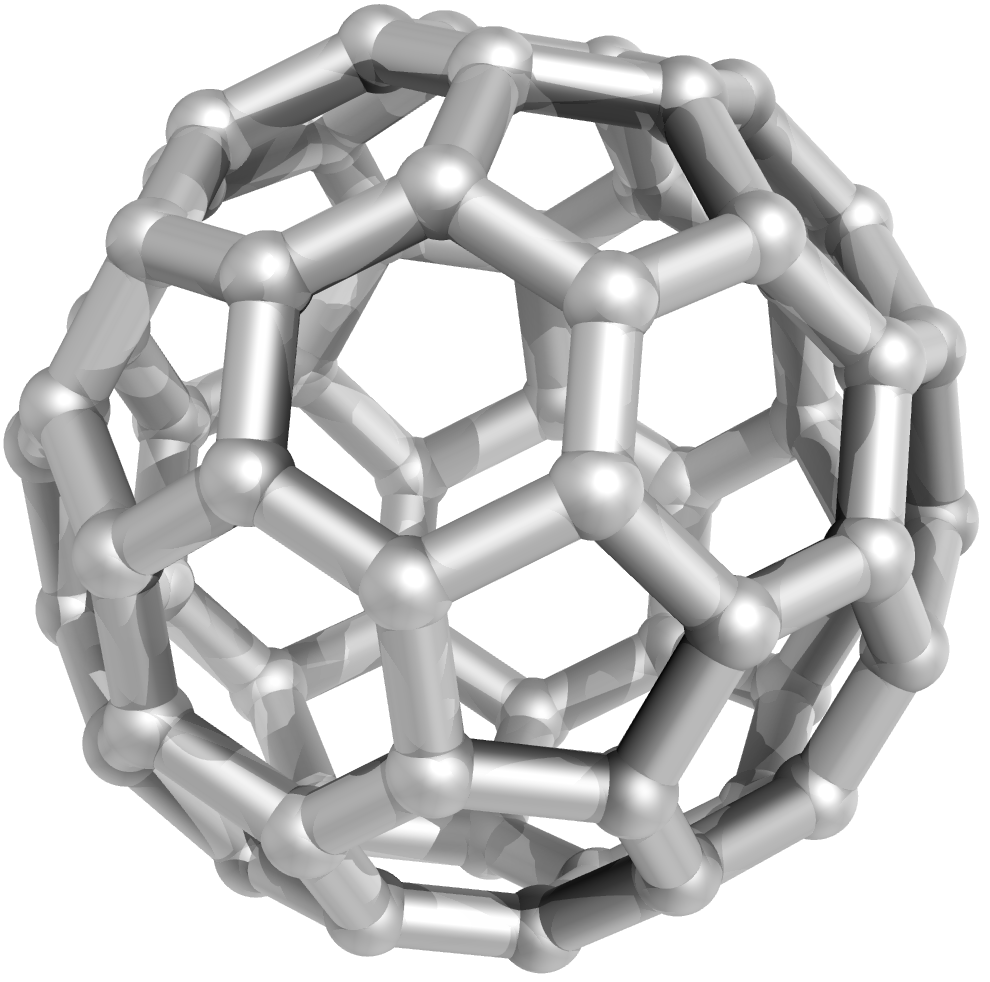
C70 fullerene is a ball-like structure with some very interesting properties and applications. Numerous computational investigations have dealt with during the last decades, for numerous purposes. Among them, an old stated concept regarding its ability to trap very fast, very energetic entities in its hollow interior space. Such entities could be high energy molecules, thermal neutrons and free radicals. Such claims are known from the 70s but had not been proved theoretically up to date. The main issue for that was the partial inefficiency of computational chemistry tools to treat sub atomic particles [in the case of neutrons] in their force fields and calculations.
Sub atomic particles’ case has many interesting fields of applications. Our client’s interest was found in the trapping of thermal neutrons from nuclear reactors. Thermal neutrons trapped in C70 fullerenes would greatly enhance the cooling [thermal transfer] process in nuclear reactors, effectively minimizing:
- The required volume of heavy water required
- The time required for steady state cooling
- The time required for cooling after shut-down [see Fukosima incident]
The mechanism of the thermal neutron trapping process is based on the thermal neutron’s high energy [kinetic] and the C70 fullerene’s hollow interior. Once the thermal neutron crosses the ‘spherical’ surface of the fullerene, it gets trapped into the hollow interior due to symmetrical interactions with its walls. Neutron will continue to move with dissipation of energy with an expected equilibrium after some ms. The overall simulation of the process has known and unknown parts. Fullerene can be designed and optimized under most commercial [and some free] codes and packages. The crossing of the fullerene boundary can also be simulated and monitored via Molecular Dynamics simulations under any field, although obviously for the specific case semi-empirical and MM+ are not appropriate; only Ab Initio or Density Functional Theory fields should be employed. The dissipation of energy inside the C70 fullerene interior can also be simulated and monitored via the same approach [MD/ DFT or MD/ Ab Initio]. The only part of the simulation process that demonstrates obstacles is the set up of the neutron model. Most commercial packages [if not all] do not include such a capability and thermal neutrons must be designed at code level.
We used pre-built neutron entities to monitor the crossing of C70 fullerene boundary and the oscillation into the C70 fullerene hollow interior. We witnessed that indeed C70 fullerene can accept, slow down and store a thermal neutron under the conditions of interest [thermal neutrons from a nuclear reactor]. It became evident that a crucial size of the fullerene exists, that would not allow for keeping the thermal neutron in its interior; this limit cannot be share here. Other structures investigated via this Computational Chemistry work included other sizes of fullerenes and nanotubes. Nanotubes are used in bundles [to maintain orientation] and are either very long [high length to diameter ratio] or closed at one cap in order to hold the thermal neutron trapped. Nanotubes exhibited lower possibility of effective collision with thermal neutrons due to the small available entrance area that allows for trapping; most nanotubes were breached from the side and depending on the diameter, neutrons were or were not trapped.